Table of Contents (click to expand)
Refracting telescopes use a convex lens to absorb and converge a larger volume of light, thereby generating a higher quality image. Reflecting telescopes use a concave mirror to reflect the rays in a converging manner, such that the converged rays reflected by the secondary mirror are further converged to form a magnified image. Spectral telescopes are dedicated to detecting a single spectrum of the entire electromagnetic spectrum.
Most of modern physics couldn’t exist if it weren’t for the invention of the telescope. Without its invention, we couldn’t have learned about the cataclysmic explosion that gave birth to the Universe, nor about how it evolved over billions of years undergoing unceasing expansion. We would never know about the existence of stellar objects inhabiting the vast expanse whose appearance transcend our perceptions of beauty and the significance of humanity in the grand scheme of the cosmos.
One day, telescopes might allow us to achieve one of the greatest of human triumphs: to find a world just like ours, perhaps inhabited by an intelligent species like us. So, how does this pivotal invention enable us to make such discoveries?
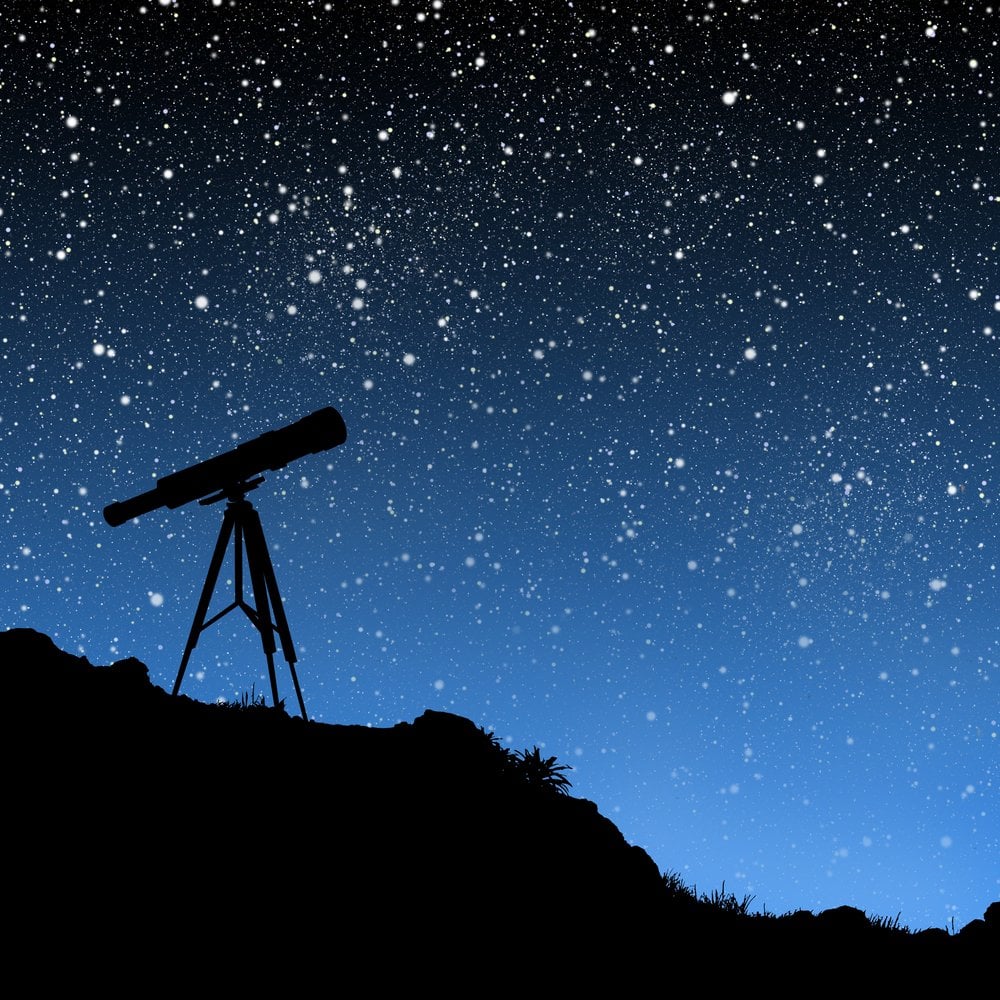
Telescopes can be categorized into three types according to the way they interact with light and the type of light they work with.
Recommended Video for you:
What Is A Refracting Telescope?
At the root of this invention are curiosity and obstinacy — curiosity to peer over distances we aren’t naturally allowed to see and obstinacy to find a way around this inadequacy. While the majority of people believe the underlying cause of our success is our tremendous ability to communicate complex ideas via language, some believe that it is simply our ability to automate – to build devices that enable us to overcome our weaknesses.
At the entrance of our eye is a type of lens that converges the light entering it towards a single point on the retina, the screen lying behind on which the images are formed. The phenomenon of light changing its direction when it enters another medium is known as refraction, while the lens that bends them inward is called a convex lens. However, our eye’s lens is so small that it can only absorb a meager amount of light that falls upon it, which makes for poor vision.
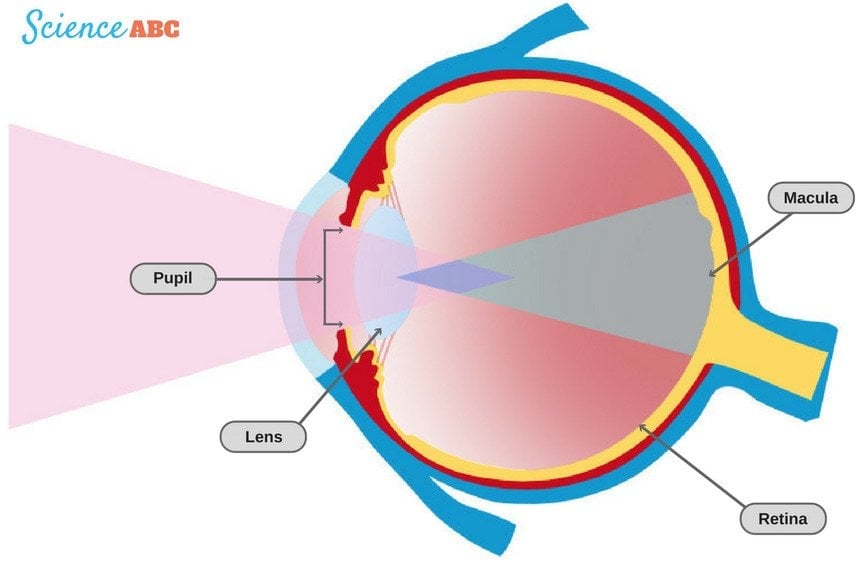
Most people associate telescopes with magnification, but while a telescope does achieve enormous magnification, a more appropriate definition is that it makes objects appear more clearly; it simply improves our vision. Now, contrary to conventional wisdom, Galileo wasn’t the one who invented the first refracting telescope, but he was very passionate and more vocal about his work than others. This self-marketing etched his name in the history books as the inventor of the first telescope. However, later, it was this very enthusiasm that cost him house arrest for life after he advocated Copernicus’ view of the Sun-in-the-center model of the Solar System.
Galileo’s principle was simple, yet highly profound: he realized that clarity was a function of the amount of light a viewing device absorbs. The looking device he built was a human eye… just larger. A refracting telescope comprises a large convex lens that absorbs and converges a larger volume of light, thereby generating a higher quality image. The area of the lens is proportional to its radius, so doubling its radius can augment the clarity by four times.
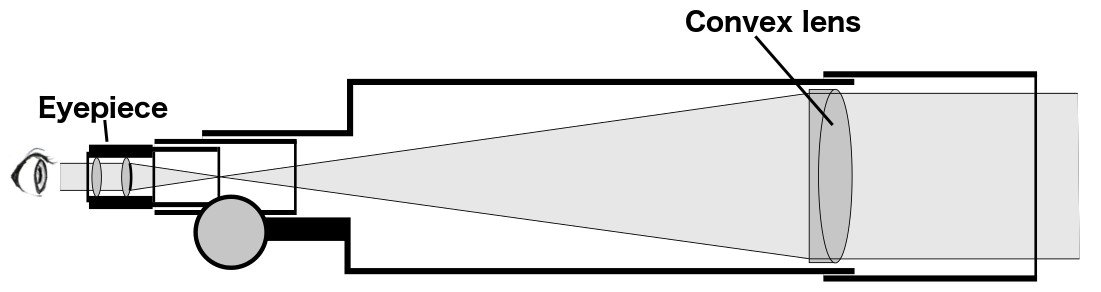
When Galileo pointed his telescope towards the sky for the first time, he couldn’t stop marveling at the moon which, for the very first time in humanity’s history, was truly exposed in all of its cratered beauty. The telescope was so powerful that he eventually discovered four moons orbiting Jupiter and the gorgeous rings of Saturn, the planet then known to be the farthest – residing twice as far away as Jupiter!
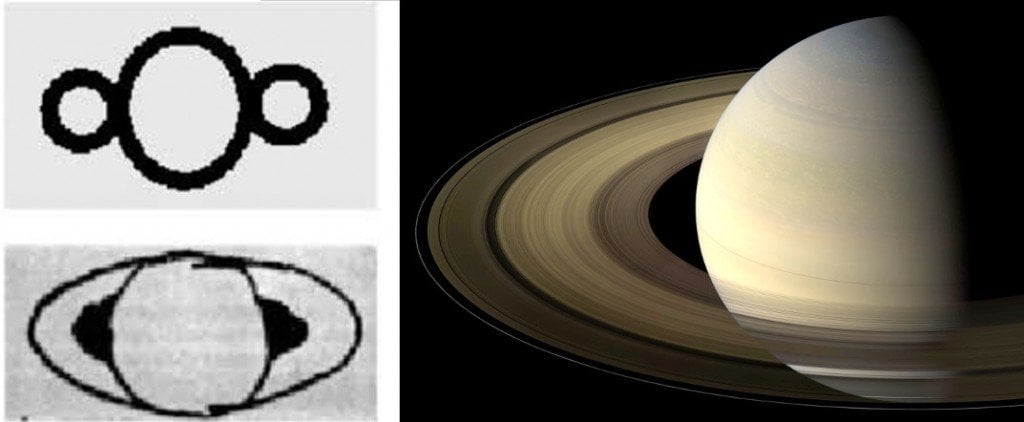
However, Saturn’s observation also revealed his telescope’s limitation. Due to the crudeness or poor resolution of his telescope, the rings appeared to be bundled together — he perceived Saturn to be a large planet squeezed between two moons. Resolution is the ability of a looking device to distinguish between two objects. While there is no limit to magnification, there is a limit to resolution. Zooming might make the object visible, but its image would be highly mushed and blurred if we demand a telescope to resolve more than its apparatus allows it to.
The limitations don’t end there — because different wavelengths of light converge at different angles, they don’t converge at a single point. This causes the image to distort or the telescope to generate inaccurate results. This is known as an aberration.
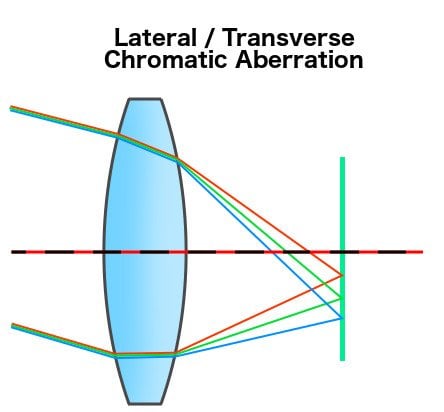
What’s more, larger lenses are thinner at their edges and thus more prone to breaking. Building them isn’t as easy as aligning lenses either. And all this hassle just to look at an inverted image! The images they produce are upside-down, which is disconcerting and takes time to adjust to.
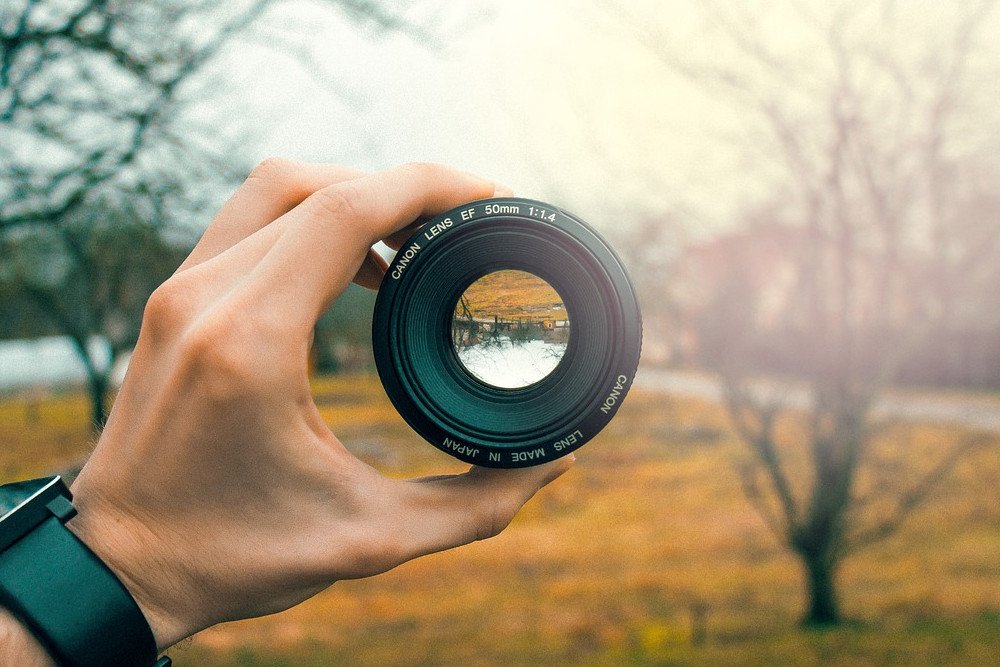
These limitations were overcome by another telescope built by a mind of equal or perhaps greater (the greatest?) intellect, none other than Sir Isaac Newton.
What Is A Reflecting Telescope?
Newton simply replaced the convex lens or, as it is called, the objective of a telescope, with a highly reflective concave mirror. The use of a mirror deterred any aberration. The shape of this mirror is parabolic, which was mathematically determined with an incentive to cause a stark inward reflection.
In Newton’s original design, when light emanating from a faraway star entered the telescope, it was reflected by the concave mirror — the primary mirror — towards a small mirror hanging in the middle — the secondary mirror — which is cleverly installed in a position to reflect the rays towards the eyepiece above. The concave mirror reflects the rays in a converging manner, such that the converged rays reflected by the secondary mirror are further converged to form a magnified image. The eyepiece comprises a lens, but because aberration is a function of a lens’ radius, the lens isn’t very large.
The design is brilliant — it is easier to build, the manufacturing cost isn’t high and only one side of the mirror must be thoroughly polished. In fact, most of our modern, highly advanced, acute telescopes are reflecting telescopes, including the Hubble telescope hovering above Earth’s atmosphere. The major limitation of reflectors, however, is their huge size. William Herschel’s telescope was one story high, big enough for him to sit inside. Also, large mirrors tend to deform at their edges, although this can be fixed with the assistance of computers.
Yet, no matter how prolific a telescope is, the incident light is bound to be distorted as it passes through the layers of our atmosphere. This is why we sent a telescope outside the atmosphere. Moreover, both refracting and reflecting telescopes are useless when we wish to observe stellar phenomena that emit light of wavelengths that lie outside the visual spectrum.
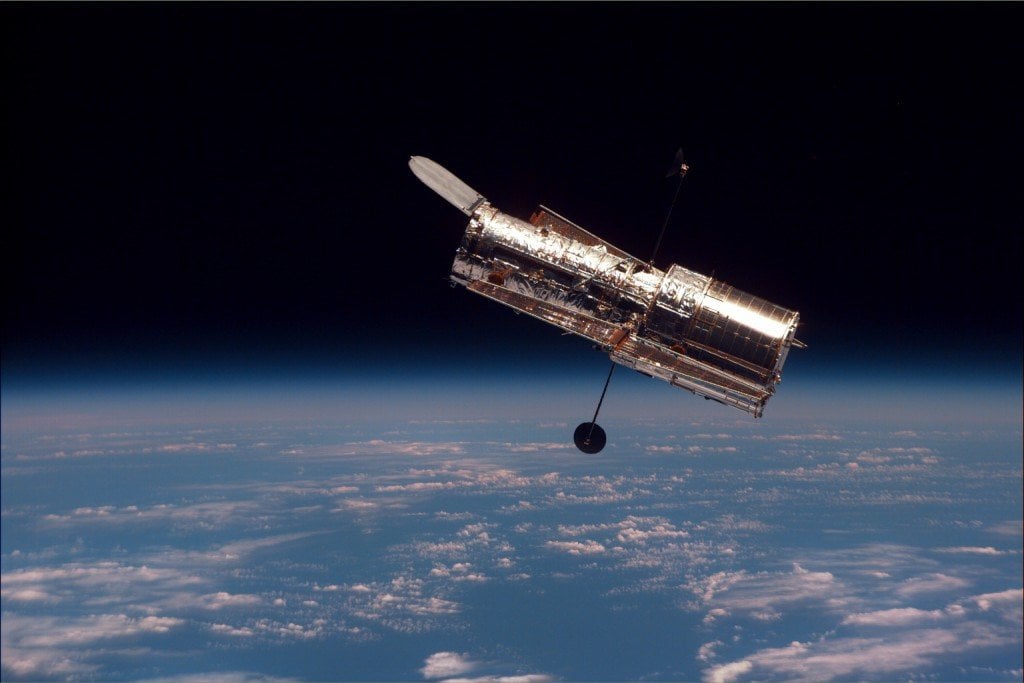
A star’s key features, such as its flares, might emit light in radio waves, which a reflecting telescope isn’t capable of capturing. The image it captures is thus incomplete, deprived of not one, but perhaps many of its other key features. Low-frequency radio waves fall around the apparatus and high-frequency gamma-rays pass straight through as if nothing was there. To solve this, we eventually developed telescopes that are dedicated to detecting a single spectrum of the entire electromagnetic spectrum.
Spectral Telescopes
The Universe is replete with a myriad of different wavelengths, so each development of spectral telescopes has been equally crucial. For instance, without infrared telescopy, we couldn’t have looked through the massive clouds of dust between us and the center of our galaxy obstructing our view of a massive object, speculated to be a black hole, one million times more massive than our Sun, around which some stars appear to revolve. Similarly, without radio telescopy, the Cosmic Background Microwave Radiation (CMBR) couldn’t have been detected. The CMBR represents one of the most groundbreaking discoveries of modern astronomy, as it is an indelible proof of the Big Bang.
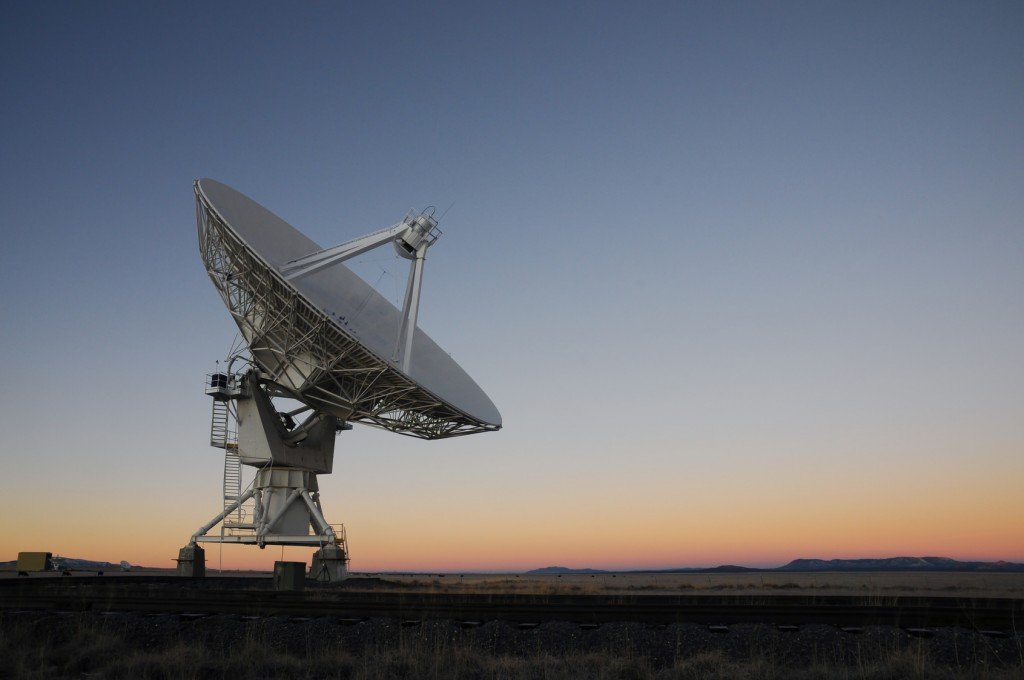
The design of a radio telescope is no different from that of a reflecting telescope. The iconic dish mimics the primary mirror, which reflects radio waves onto a small secondary plate above it, which in turn converges them towards the receiver installed beneath the dish, at its center. The radio information is converted into color information by a computer. These telescopes are particularly lucrative because radio waves are the least affected by the atmosphere, so astronomers can observe their favorite stars from Earth without any difficulty.
What’s more, they produce ridiculously high-resolution images when a group of them are aligned together in a certain way. You will find such a family of gigantic dishes secluded in pollution-free deserts. This is known as interferometry. When aligned in a certain way, each telescope produces the image with its own perspective, such that an extremely high-resolution, distortion-free, colored image is created when every perspective is combined.
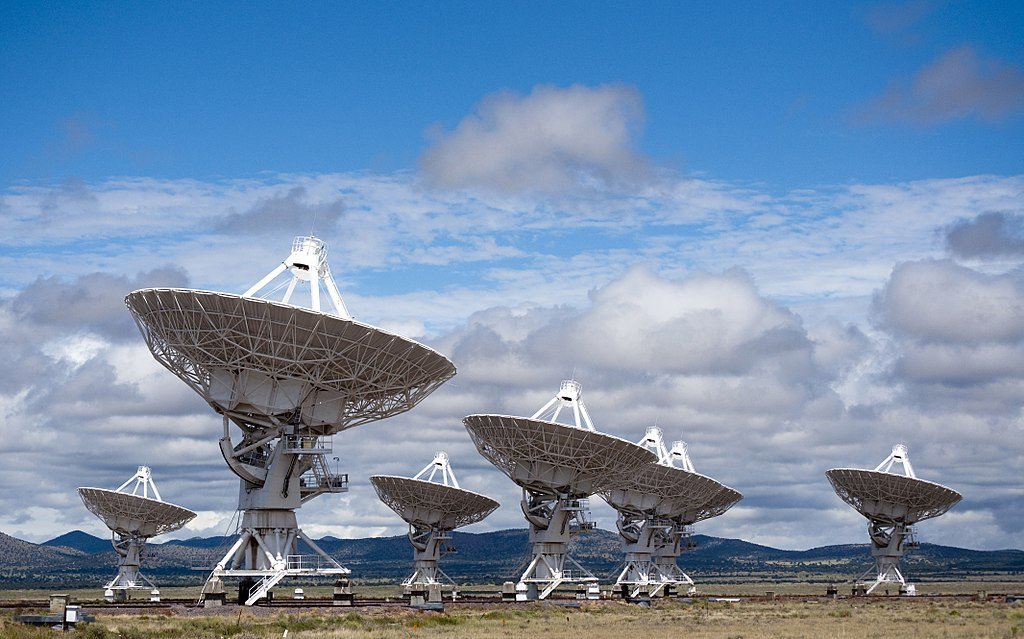
Similarly, there are intricate telescopes that detect all types of radiation, such as gamma-rays, which are emitted by supernovae – the tremendously explosive deaths of stars, and X-rays, without which we couldn’t have confirmed the existence of black holes, which were first predicted mathematically.
Hubble Deep Field
Humans are hardwired to observe, but we always, for it is logical, point our gaze at a target. However, back in 1995, the Hubble telescope hovering above us was commissioned to turn its gaze towards…. nothing. The telescope was pointed at a tiny region in space in Ursa Major that was analogous to the gap between leaves that allows a ray of sunlight through into the forest. The region was chosen on purpose for it was found to be empty, and therefore would allow light from faraway galaxies to pierce through. It had to be guaranteed that nothing bright was in the way. The telescope would then look at stars far beyond our galaxy, unhindered.
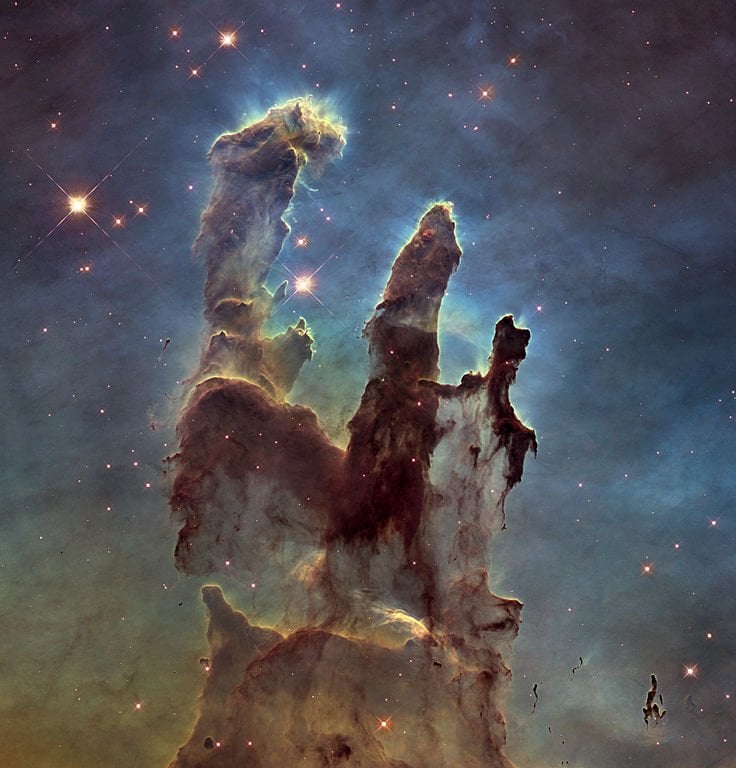
The angle that a telescope draws to observe the moon from the Earth is around half a degree or 30 arcminutes (1 degree = 60 arcminutes). However, the Hubble telescope was peeping through a region that would isolate a cone of merely 2.6 arcminutes, which is comparable to the thin region a pin’s head occupies when stretched at arm’s length against the sky!
NASA observed the region for 10 days and what they found blew their minds. The 3,000 galaxies captured in the Hubble Deep Field were light-years away from us, so, the light reaching us pictured them not as they were then but how they appeared a few billion years ago. The telescope had peeped into the past, discovering ‘fossils’ and layers of images, each recording our Universe’s progressive evolution over a few billion years. It was as if the telescope had pointed towards the Earth and photographed the earliest Homo sapiens leaving their caves in the background, then the Egyptians building the pyramids and finally Galileo observing Saturn.
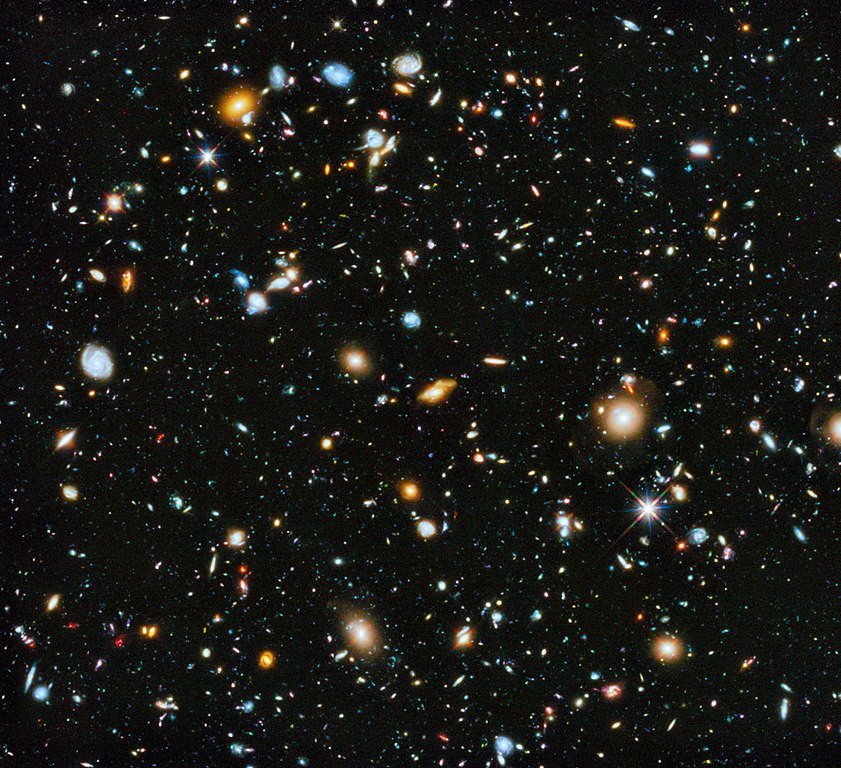
Hubble had photographed stars formed as early as 12.2 billion years ago, just one billion years short of the Big Bang. A year later, to test whether this spot wasn’t particularly special, they pointed it towards the south and produced the Hubble Deep Field South, a similar image that captured the evolution of younger galaxies. After almost 15 years, NASA installed an infrared camera and photographed stars that were born right after the ‘Dark Ages’, the time following the Big Bang when temperatures plummeted and matter began to coalesce. The Hubble Ultra Deep Field is the deepest portrait of the Universe mankind has ever captured. The invention of the telescope allowed us to travel in time, almost to its very beginning.