Table of Contents (click to expand)
The study of antimatter provides insights into understanding the symmetry between matter and antimatter, which is a crucial aspect of particle physics.
Antimatter has fascinated scientists and science fiction enthusiasts for decades. While it possesses extraordinary properties and has the potential to revolutionize various fields, there’s one challenge that has confused scientists for years—antimatter is incredibly short-lived.
So, why do scientists continue to create something that vanishes almost instantly?
In 1928, physicist Paul Dirac proposed an idea, namely that for every particle of matter in the universe, there should exist an antimatter counterpart.
However, shortly after the Big Bang, a mysterious cosmic event occurred, resulting in the disappearance of most antimatter, leaving behind the less than 5% of matter that constitutes the universe as we know it today.
Recommended Video for you:
Introduction To Antimatter
According to modern physics, every particle of matter has a corresponding antiparticle. For example, the antiparticle of an electron is called a positron, which carries a positive charge.
When matter and antimatter particles meet, they annihilate each other, releasing energy in the process.
This annihilation in mathematical terms is given by Einstein’s famous equation, E=mc², where E represents energy, m is mass, and c is the speed of light. The result of this equation is a burst of energy that’s tremendously efficient.
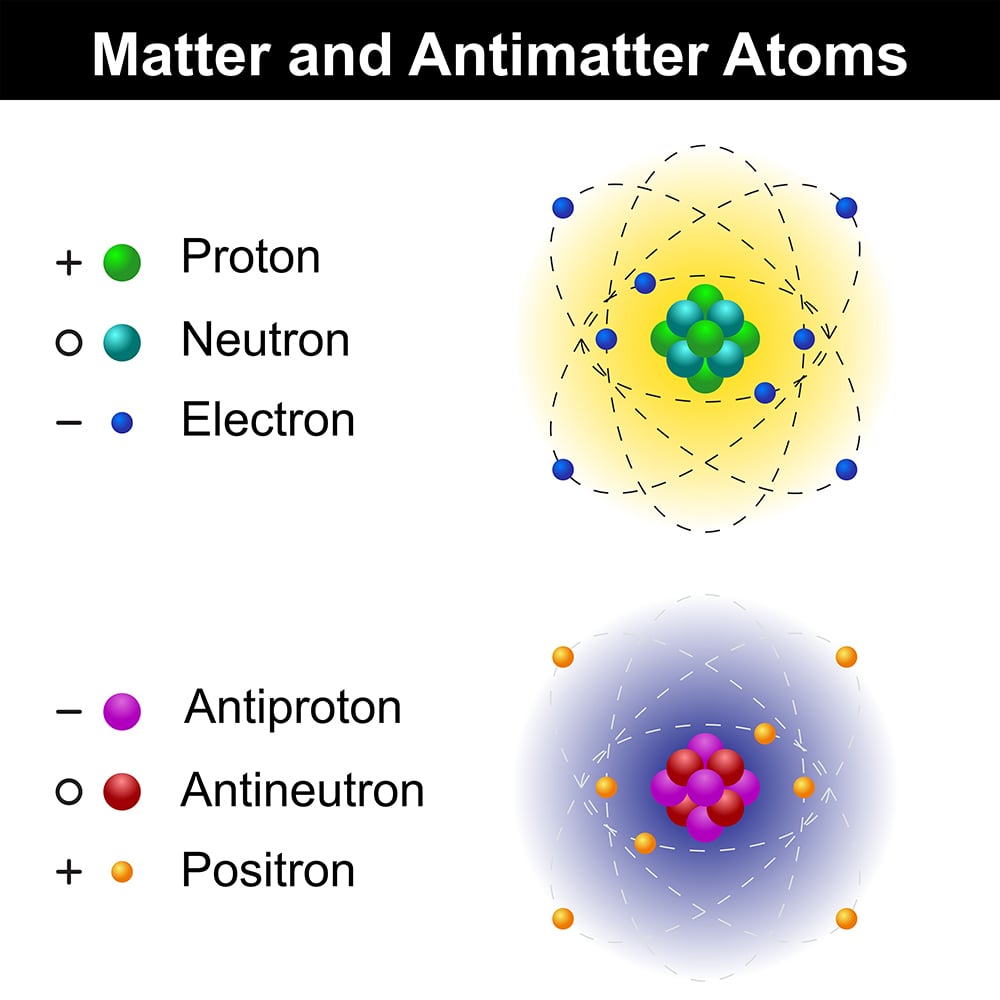
The energy release from matter-antimatter annihilation is far more potent than anything achievable through chemical reactions, nuclear fission, or even nuclear fusion. It is the energy source of the future that has fueled countless narratives (in the movies, of course), from spacecraft propulsion to the ultimate power source.
In theory, just a tiny amount of antimatter could produce an immense amount of energy, making it a potential game-changer for space exploration and energy generation on Earth.
The Strange Case Of Antihydrogen
The simplest atom in the universe is hydrogen, consisting of a single proton and an orbiting electron. Antimatter’s counterpart to this fundamental element is antihydrogen, a neutral atom composed of a positively charged positron orbiting around a negatively charged antiproton.
In 1995, physicists at the European Organization for Nuclear Research (CERN) achieved a groundbreaking milestone by successfully creating the first antihydrogen atoms.
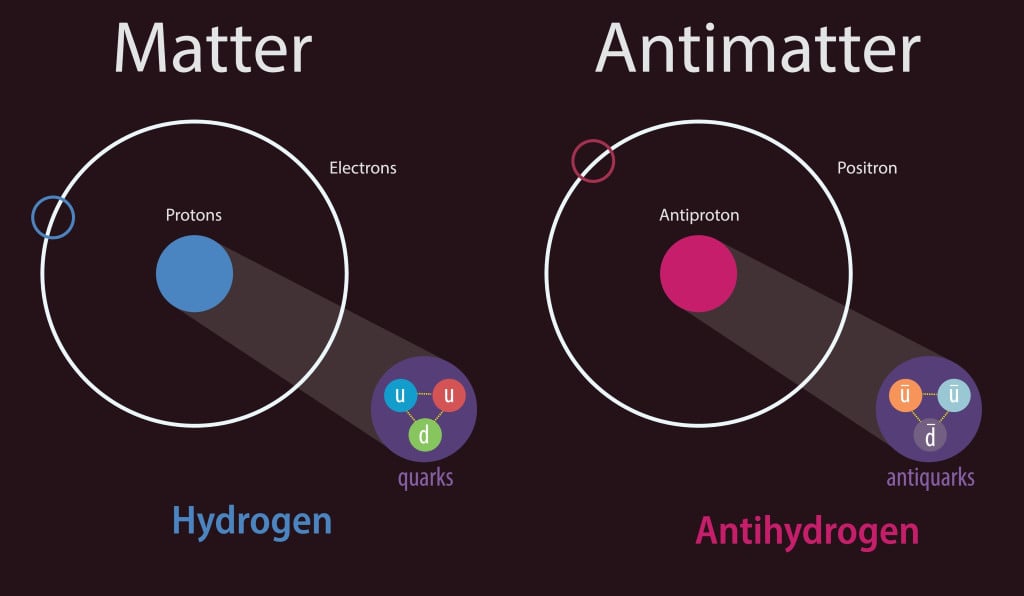
This was accomplished by the CERN’s Low Energy Antiproton Ring (LEAR). The process involved allowing energetic antiprotons to collide with heavy atomic nuclei, a collision that could produce electron-positron pairs.
After producing an actual piece of Antimatter, trapping it for longer periods of time was the essential task for the scientists. It was difficult, but essential, to store it for effective studying of this antiparticle’s behavior.
The solution came in the form of the Antiproton Decelerator, established at CERN in the late 1990s, which provided slower-moving, lower-energy antiprotons for antimatter experiments. Silicon detectors are employed to detect and locate the strong bursts of energy, confirming the presence of trapped antihydrogen.
One of the primary objectives of the ALPHA experiment at CERN in 2011 was to conduct precise comparisons between hydrogen and antihydrogen to determine if they share the same spectral lines, a critical test of the fundamental symmetry between matter and antimatter.
For this, scientists successfully stored antimatter atoms for about 16 minutes. With this new ability to trap antihydrogen for extended periods, researchers now have the opportunity to understand its properties in greater detail.
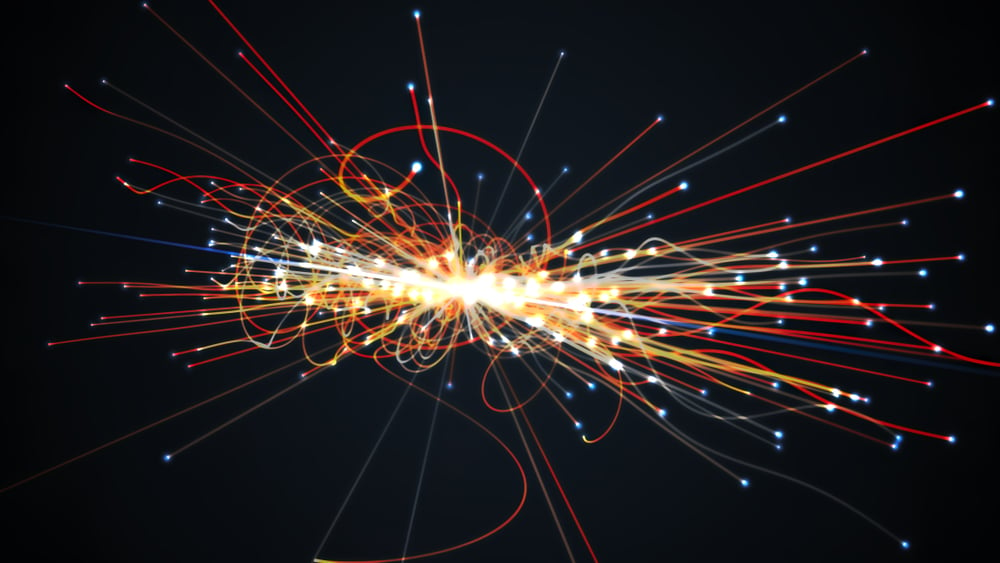
Another research endeavor—the AEGIS experiment—aims to measure the gravitational acceleration constant (g) as experienced by antihydrogen atoms. These experiments, among others, hold the potential to unlock the mysteries surrounding antimatter.
Now, if antimatter is so challenging to create and store, why do scientists persist in their efforts?
The answer lies in the pursuit of knowledge and to answer fundamental questions about the universe. Antimatter is not just a potential energy source; it’s a fundamental aspect of particle physics and cosmology. It provides insights into the symmetry between matter and antimatter and the stubborn mystery of why there is more matter than antimatter in the universe.
Medical And Practical Applications Of Antimatter
Antimatter isn’t just about powering starships; it has practical and realistic applications too! In the field of medicine, positron emission tomography (PET) scans rely on antimatter. Positrons are used to create images of the human body, aiding in the diagnosis and treatment of various diseases. This medical technology has saved countless lives.
While the idea of antimatter propulsion remains largely theoretical, it’s an area of active research. The concept involves using the energy released from matter-antimatter annihilation as a highly efficient propulsion method for spacecraft.
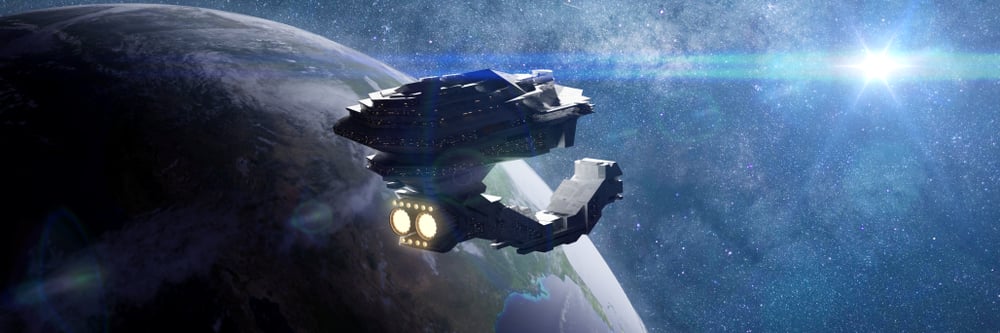
In conclusion, the creation and study of antimatter persist as areas of intense scientific interest and inquiry. Despite its nature and the difficulties associated with containment and production, antimatter research holds the promise of profound insights into the fundamental nature of the universe.