Table of Contents (click to expand)
By definition, oxidative phosphorylation is the process by which electrons from NADH and FADH2 are transferred to O2 molecules through a series of electron carriers/protein complexes in order to generate ATP from ADP for the cell’s energetic needs.
As many of you know, creating usable energy is one of the most important functions of nearly every cell in the human body. That “usable energy” comes in the form of ATP (adenosine triphosphate), and is generated through cellular respiration, a series of metabolic pathways that can include both aerobic (in the presence of oxygen) and anaerobic (lacking oxygen) processes.
In cellular respiration, there are four main stages – glycolysis, fatty acid oxidation, the Krebs’ Cycle (or Citric Acid Cycle), and the electron transport chain. The first three stages are explained in detail in this article. The final stage, however, the electron transport chain, is where oxidative phosphorylation occurs, and also where the vast majority of energy is harnessed during the vital process of cellular respiration.
Recommended Video for you:
What Is Oxidative Phosphorylation?
By definition, oxidative phosphorylation is the process by which electrons from NADH and FADH2 are transferred to O2 molecules through a series of electron carriers/protein complexes in order to generate ATP from ADP for the cell’s energetic needs. That definition may be concise, but the steps through which this energy generation occurs is not nearly as simple. Understanding the intricacy of oxidative phosphorylation (the electron transport chain) was a major challenge in biochemistry for decades. In fact, it took researchers more than a century to accurately place all the pieces of cellular respiration.
The Process Of Oxidative Phosphorylation
The raw materials in oxidative phosphorylation are the NADH and the FADH2 molecules that have been generated during glycolysis and the Krebs’ Cycle. The events of oxidative phosphorylation occur in the mitochondria, more specifically in 5 types of protein complexes that bridge the space between the mitochondrial matrix and the intermembrane space. These protein complexes are electron carriers, and some also act as ion pumps. Oxidative phosphorylation can be divided into two basic parts, the oxidation steps and the energy-generation events.
Oxidation Reactions
At the start of the electron transport chain, NADH and FADH2 are present inside the mitochondria, both of which carry high-energy molecules. NADH drops off its high-energy molecules (H+) in an oxidation reaction to Protein Complex 1, while FADH2 loses its electrons during a similar oxidation reaction in Protein Complex 2. NADH becomes NAD+ and FADH2 becomes FAD. Basically, the energy of the electrons is used to pump the protons (H+) into the intermembrane space, away from the mitochondrial matrix. This will create a gradient, i.e., a higher concentration of protons in the intermembrane space than inside the mitochondria. This gradient functions as a temporary storehouse for transformed energy before it can be utilized.
The electrons lost during the oxidation reaction of NADH and FADH2 are then transferred to Protein Complex 3 along the electron transport chain, aided by Coenzyme Q. Protein Complex 3 is another ion pump, using the electrons to pump more hydrogen ions out of the mitochondria. Cytochrome C then transfers electrons from Protein Complex 3 to Protein Complex 4.
After moving through numerous protein complexes and fueling various reactions, the electrons are at a much lower energy level by the time they finish moving through Protein Complex 4. At this point, the electrons combine with protons and oxygen in the mitochondria to form water (H2O). This is a reduction reaction, as opposed to the oxidation reactions that occurred in the previous protein complexes. Oxygen is the last electron acceptor in oxidative phosphorylation. This entire process is a part of aerobic respiration, meaning that oxygen must be present. Without oxygen, none of the steps outlined above would have occurred.
Energy Generation
Now that the electrons have been used to push protons out into the intermembrane space, an electrochemical gradient has been established. There is a higher positive charge on the outside of the mitochondria than there is on the inside. This gradient will be the final catalyst that allows for usable energy generation through oxidative phosphorylation.
The final protein complex involved in oxidative phosphorylation is ATP synthase, which is essentially a motor or factory that creates ATP, the basic unit of energy for all metabolic processes and chemical pathways – the fuel for our cellular survival. The protons that have accumulated on the outside of the mitochondrial membrane now move through the ATP synthase, which spins as the protons pass through. This final step – where specific molecules are allowed to selectively pass back through the ATP synthase – is referred to as chemiosmosis. This movement of protons will enable ADP to bind another inorganic phosphorous molecule, resulting in the formation of ATP! The binding of a phosphorous group to any other molecule is known as phosphorylation.
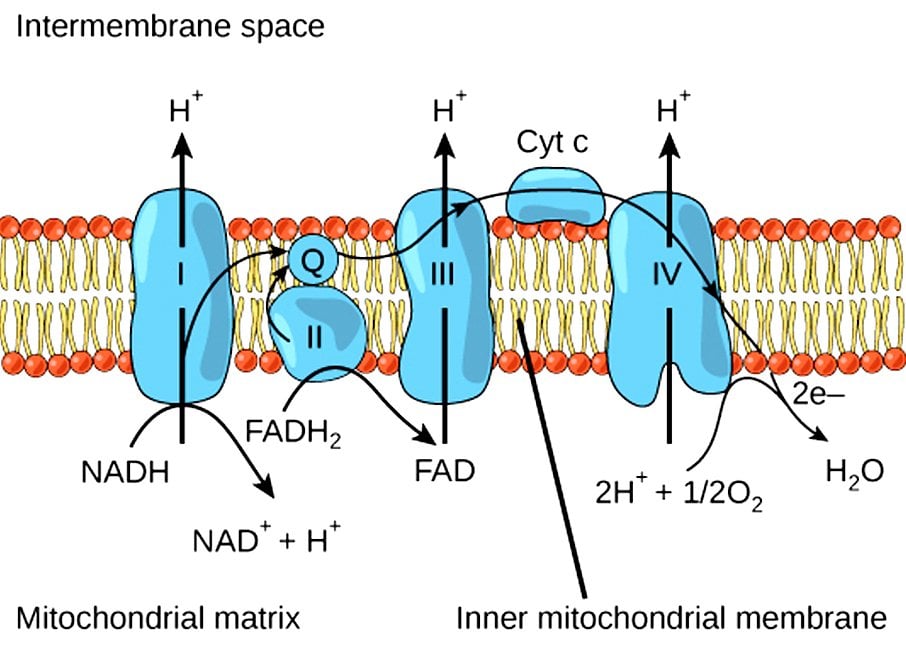
Thus, when you look at the title of this article, and the name of this critical process, it makes a lot of sense! Oxidative reactions create the proton gradient and use the energy derived from electrons, while phosphorylation is the last step that creates usable energy.
The Dark Side Of Oxidative Phosphorylation?
While this final step in cellular respiration is a critical one in eukaryotes, it also generates a number of reactive oxygen species. When health professionals talk about oxidative stress and free radicals, they are referring to some of the end products and results of oxidative phosphorylation. The production of such substances, i.e., Hydrogen peroxide and superoxide, are unavoidable in the body, and can lead to an increased risk of cancer, inflammation and chronic disease. To counter this damage, antioxidants can be found in a wide variety of foods, particularly fruits and vegetables.
A Final Word
Oxidative phosphorylation is the most efficient means of generating energy in cells, but it is not the only method. Substrate phosphorylation, for example, occurs in glycolysis and the Krebs’ Cycle, both of which generate two molecules of ATP, without relying on chemiosmosis or proton gradients. Furthermore, in the absence of oxygen, energy can be generated through fermentation or anaerobic respiration, although both of these processes are less efficient and may produce unwanted byproducts, such as lactic acid.
Finally, the process of oxidative phosphorylation only occurs in eukaryotes; prokaryotes, on the other hand, such as bacteria, lack mitochondria, but can create a similar gradient in their own plasma membrane in order to generate ATP. You can learn more about the details of these anaerobic processes in this article.